A supernova caused the BOAT gamma ray burst, JWST data confirms
Still the BOAT —
But astronomers are puzzled by the lack of signatures of expected heavy elements.
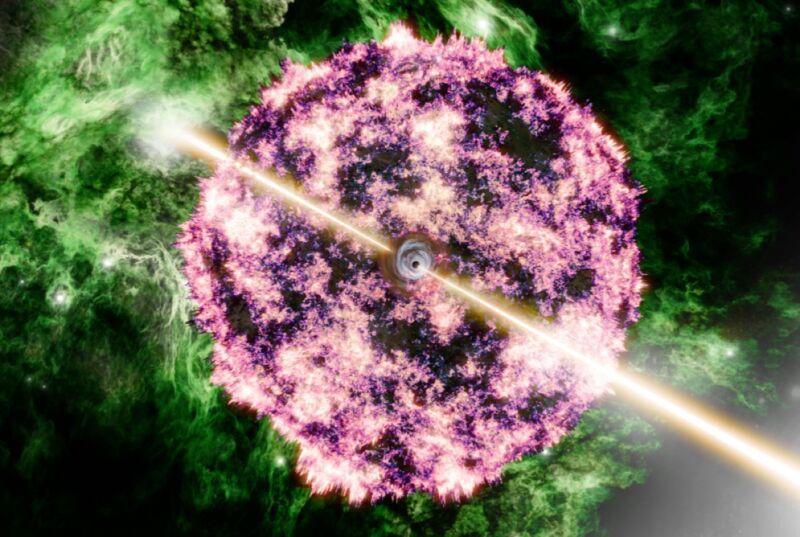
Enlarge / Artist’s visualization of GRB 221009A showing the narrow relativistic jets—emerging from a central black hole—that gave rise to the brightest gamma-ray burst yet detected.
Aaron M. Geller/Northwestern/CIERA/ ITRC&DS
In October 2022, several space-based detectors picked up a powerful gamma-ray burst so energetic that astronomers nicknamed it the BOAT (Brightest Of All Time). Now they’ve confirmed that the GRB came from a supernova, according to a new paper published in the journal Nature Astronomy. However, they did not find evidence of heavy elements like platinum and gold one would expect from a supernova explosion, which bears on the longstanding question of the origin of such elements in the universe.
As we’ve reported previously, gamma-ray bursts are extremely high-energy explosions in distant galaxies lasting between mere milliseconds to several hours. There are two classes of gamma-ray bursts. Most (70 percent) are long bursts lasting more than two seconds, often with a bright afterglow. These are usually linked to galaxies with rapid star formation. Astronomers think that long bursts are tied to the deaths of massive stars collapsing to form a neutron star or black hole (or, alternatively, a newly formed magnetar). The baby black hole would produce jets of highly energetic particles moving near the speed of light, powerful enough to pierce through the remains of the progenitor star, emitting X-rays and gamma rays.
Those gamma-ray bursts lasting less than two seconds (about 30 percent) are deemed short bursts, usually emitting from regions with very little star formation. Astronomers think these gamma-ray bursts are the result of mergers between two neutron stars, or a neutron star merging with a black hole, comprising a “kilonova.” That hypothesis was confirmed in 2017 when the LIGO collaboration picked up the gravitational wave signal of two neutron stars merging, accompanied by the powerful gamma-ray bursts associated with a kilonova.
The October 2022 gamma-ray burst falls into the long category, lasting over 300 seconds. GRB 221009A triggered detectors aboard NASA’s Fermi Gamma-ray Space Telescope, the Neil Gehrels Swift Observatory, and Wind spacecraft, among others, just as gamma-ray astronomers had gathered for an annual meeting in Johannesburg, South Africa. The powerful signal came from the constellation Sagitta, traveling some 1.9 billion years to Earth.
Several papers were published last year reporting on the analytical results of all the observational data. Those findings confirmed that GRB 221009A was indeed the BOAT, appearing especially bright because its narrow jet was pointing directly at Earth. But the various analyses also yielded several surprising results that puzzled astronomers. Most notably, a supernova should have occurred a few weeks after the initial burst, but astronomers didn’t detect one, perhaps because it was very faint, and thick dust clouds in that part of the sky were dimming any incoming light.
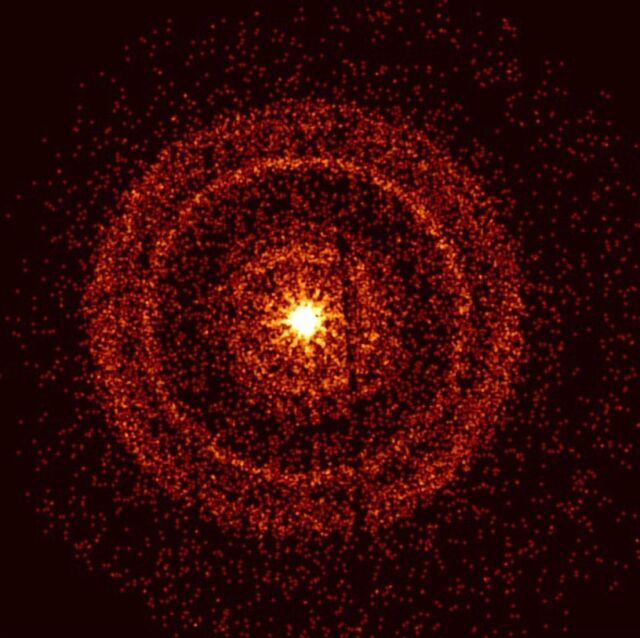
Enlarge / Swift’s X-ray Telescope captured the afterglow of GRB 221009A about an hour after it was first detected.
NASA/Swift/A. Beardmore (University of Leicester)
That’s why Peter Blanchard of Northwestern University and his fellow co-authors decided to wait six months before undertaking their own analysis, relying on data collected during the GRB’s later phase by the Webb Space Telescope’s Near Infrared Spectrograph. They augmented that spectral data with observations from ALMA (Atacama Large Millimeter/Submillimeter Array) in Chile so they could separate light from the supernova and the GRB afterglow. The most significant finding was the telltale signatures of key elements like calcium and oxygen that one would expect to find with a supernova.
Yet the supernova wasn’t brighter than other supernovae associated with less energetic GRBs, which is puzzling. “You might expect that the same collapsing star producing a very energetic and bright GRB would also produce a very energetic and bright supernova,” said Blanchard. “But it turns out that’s not the case. We have this extremely luminous GRB, but a normal supernova.” The authors suggest that this might have something to do with the shape and structure of the relativistic jet, which was much narrower than other GRB jets, resulting in a more focused and brighter beam of light.
The data held another surprise for astronomers. The only confirmed source of heavy elements in the universe to date is the merging of binary neutron stars. But per Blanchard, there are far too few neutron star mergers to account for the abundance of heavy elements, so there must be another source. One hypothetical additional source is a rapidly spinning massive star that collapses and explodes into a supernova. Alas, there was no evidence of heavy elements in the JWST spectral data regarding the BOAT.
“When we confirmed that the GRB was generated by the collapse of a massive star, that gave us the opportunity to test a hypothesis for how some of the heaviest elements in the universe are formed,” said Blanchard. “We did not see signatures of these heavy elements, suggesting that extremely energetic GRBs like the BOAT do not produce these elements. That doesn’t mean that all GRBs do not produce them, but it’s a key piece of information as we continue to understand where these heavy elements come from. Future observations with JWST will determine if the BOAT’s ‘normal’ cousins produce these elements.”
Nature Astronomy, 2024. DOI: 10.1038/s41550-024-02237-4 (About DOIs).
A supernova caused the BOAT gamma ray burst, JWST data confirms Read More »