Armada to Apophis—scientists recycle old ideas for rare asteroid encounter
Tick-tock —
“It will miss the Earth. It will miss the Earth. It will miss the Earth.”
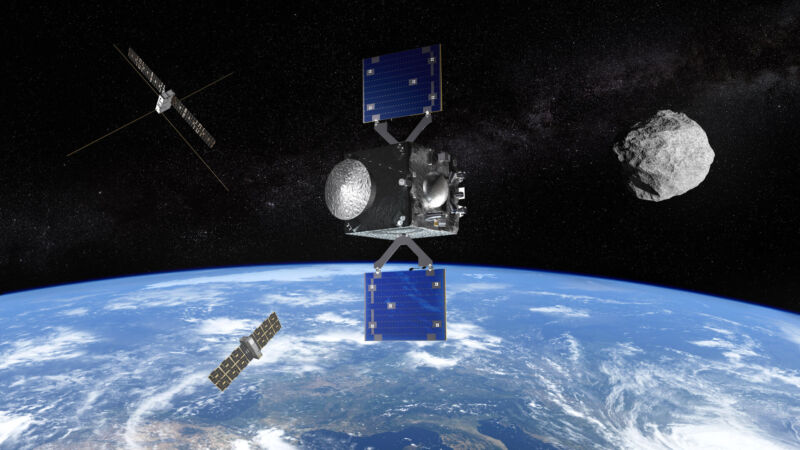
Enlarge / This artist’s concept shows the possible appearance of ESA’s RAMSES spacecraft, which will release two small CubeSats for additional observations at Apophis.
For nearly 20 years, scientists have known an asteroid named Apophis will pass unusually close to Earth on Friday, April 13, 2029. But most officials at the world’s space agencies stopped paying much attention when updated measurements ruled out the chance Apophis will impact Earth anytime soon.
Now, Apophis is again on the agenda, but this time as a science opportunity, not as a threat. The problem is there’s not much time to design, build and launch a spacecraft to get into position near Apophis in less than five years. The good news is there are designs, and in some cases, existing spacecraft, that governments can repurpose for missions to Apophis, a rocky asteroid about the size of three football fields.
Scientists discovered Apophis in 2004, and the first measurements of its orbit indicated there was a small chance it could strike Earth in 2029 or in 2036. Using more detailed radar observations of Apophis, scientists in 2021 ruled out any danger to Earth for at least the next 100 years.
“The three most important things about Apophis are: It will miss the Earth. It will miss the Earth. It will miss the Earth,” said Richard Binzel, a professor of planetary science at MIT. Binzel has co-chaired several conferences since 2020 aimed at drumming up support for space missions to take advantage of the Apophis opportunity in 2029.
“An asteroid this large comes this close only once per 1,000 years, or less frequently,” Binzel told Ars. “This is an experiment that nature is doing for us, bringing a large asteroid this close, such that Earth’s gravitational forces and tidal forces are going to tug and possibly shake this asteroid. The asteroid’s response is insightful to its interior.”
It’s important, Binzel argues, to get a glimpse of Apophis before and after its closest approach in 2029, when it will pass less than 20,000 miles (32,000 kilometers) from Earth’s surface, closer than the orbits of geostationary satellites.
“This is a natural experiment that will reveal how hazardous asteroids are put together, and there is no other way to get this information without vastly complicated spacecraft experiments,” Binzel said. “So this is a once-per-many-thousands-of-years experiment that nature is doing for us. We have to figure out how to watch.”
This week, the European Space Agency announced preliminary approval for a mission named RAMSES, which would launch in April 2028, a year ahead of the Apophis flyby, to rendezvous with the asteroid in early 2029. If ESA member states grant full approval for development next year, the RAMSES spacecraft will accompany Apophis throughout its flyby with Earth, collecting imagery and other scientific measurements before, during, and after closest approach.
The challenge of building and launching RAMSES in less than four years will serve as good practice for a potential future real-world scenario. If astronomers find an asteroid that’s really on a collision course with Earth, it might be necessary to respond quickly. Given enough time, space agencies could mount a reconnaissance mission, and if necessary, a mission to deflect or redirect the asteroid, likely using a technique similar to the one demonstrated by NASA’s DART mission in 2022.
“RAMSES will demonstrate that humankind can deploy a reconnaissance mission to rendezvous with an incoming asteroid in just a few years,” said Richard Moissl, head of ESA’s planetary defense office. “This type of mission is a cornerstone of humankind’s response to a hazardous asteroid. A reconnaissance mission would be launched first to analyze the incoming asteroid’s orbit and structure. The results would be used to determine how best to redirect the asteroid or to rule out non-impacts before an expensive deflector mission is developed.”
Shaking off the cobwebs
In order to make a 2028 launch feasible for RAMSES, ESA will reuse the design of a roughly half-ton spacecraft named Hera, which is scheduled for launch in October on a mission to survey the binary asteroid system targeted by the DART impact experiment in 2022. Copying the design of Hera will reduce the time needed to get RAMSES to the launch pad, ESA officials said.
“Hera demonstrated how ESA and European industry can meet strict deadlines and RAMSES will follow its example,” said Paolo Martino, who leads ESA’s development of Ramses, which stands for the Rapid Apophis Mission for Space Safety.
ESA’s space safety board recently authorized preparatory work on the RAMSES mission using funds already in the agency’s budget. OHB, the German spacecraft manufacturer that is building Hera, will also lead the industrial team working on RAMSES. The cost of RAMSES will be “significantly lower” than the 300-million-euro ($380 million) cost of the Hera mission, Martino wrote in an email to Ars.
“There is still so much we have yet to learn about asteroids but, until now, we have had to travel deep into the Solar System to study them and perform experiments ourselves to interact with their surface,” said Patrick Michel, a planetary scientist at the French National Center for Scientific Research, and principal investigator on the Hera mission.
“For the first time ever, nature is bringing one to us and conducting the experiment itself,” Michel said in a press release. “All we need to do is watch as Apophis is stretched and squeezed by strong tidal forces that may trigger landslides and other disturbances and reveal new material from beneath the surface.”
Assuming it gets the final go-ahead next year, RAMSES will join NASA’s OSIRIS-APEX mission in exploring Apophis. NASA is steering the spacecraft, already in space after its use on the OSIRIS-REx asteroid sample return mission, toward a rendezvous with Apophis in 2029, but it won’t arrive at its new target until a few weeks after its close flyby of Earth. The intricacies of orbital mechanics prevent a rendezvous with Apophis any earlier.
Observations from OSIRIS-APEX, a larger spacecraft than RAMSES with a sophisticated suite of instruments, “will deliver a detailed look of what Apophis is like after the Earth encounter,” Binzel said. “But until we establish the state of Apophis before the Earth encounter, we have only one side of the picture.”
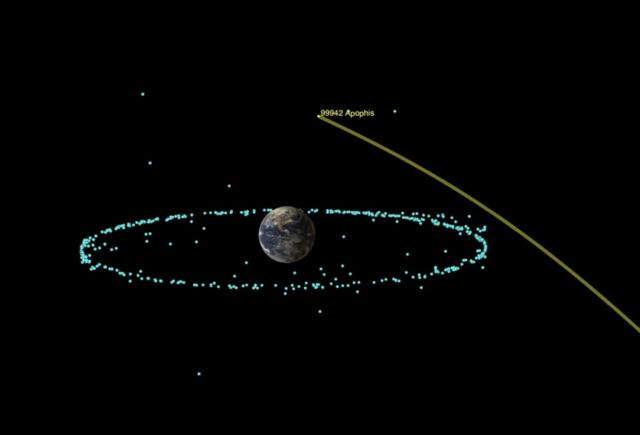
Enlarge / At its closest approach, asteroid Apophis will closer to Earth than the ring of geostationary satellites over the equator.
Scientists are also urging NASA to consider launching a pair of mothballed science probes on a trajectory to fly by Apophis some time before its April 2029 encounter with Earth. These two spacecraft were built for NASA’s Janus mission, which the agency canceled last year after the mission fell victim to launch delays with NASA’s larger Psyche asteroid explorer. The Janus probes were supposed to launch on the same rocket as Psyche, but problems with the Psyche mission forced a delay in the launch of more than one year.
Despite the delay, Psyche could still reach its destination in the asteroid belt, but the new launch trajectory meant Janus would be unable to visit the two binary asteroids scientists originally wanted to explore with the probes. After spending nearly $50 million on the mission, NASA put the twin Janus spacecraft, each about the size of a suitcase, into long-term storage.
At the most recent workshop on Apophis missions in April, scientists heard presentations on more than 20 concepts for spacecraft and instrument measurements at Apophis.
They included an idea from Blue Origin, Jeff Bezos’s space company, to use its Blue Ring space tug as a host platform for multiple instruments and landers that could descend to the surface of Apophis, assuming research institutions have enough time and money to develop their payloads. A startup named Exploration Laboratories has proposed partnering with NASA’s Jet Propulsion Laboratory on a small spacecraft mission to Apophis.
“At the conclusion of the workshop, it was my job to try to bring forward some consensus, because if we don’t have some consensus on our top priority, we may end up with nothing,” Binzel said. “The consensus recommendation for ESA was to more forward with RAMSES.”
Workshop participants also gently nudged NASA to use the Janus probes for a mission to Apophis. “Apophis is a mission in search of a spacecraft, and Janus is a spacecraft in search of a mission,” Binzel said. “As a matter of efficiency and basic logic, Janus to Apophis is the highest priority.”
A matter of money
But NASA’s science budget, and especially funding for its planetary science vision, is under stress. Earlier this week, NASA canceled an already-built lunar rover named VIPER after spending $450 million on the mission. The mission had exceeded its original development cost by greater than 30 percent, prompting an automatic cancellation review.
The funding level for NASA’s science mission directorate this year is nearly $500 million less than last year’s budget, and $900 million below the White House’s budget request for fiscal year 2024. Because of the tight budget, NASA officials have said, for now, they are not starting development of any new planetary science missions as they focus on finishing projects already in the pipeline, like the Europa Clipper mission, the Dragonfly quadcopter to visit Saturn’s moon Titan, and the Near-Earth Object (NEO) Surveyor telescope to search for potentially hazardous asteroids.
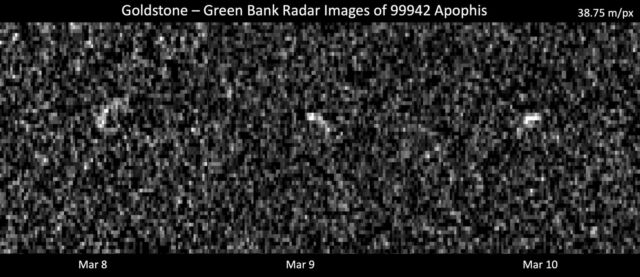
Enlarge / These grainy radar views of asteroid Apophis were captured using radars at NASA’s Goldstone Deep Space Communications Complex in California and Green Bank Telescope in West Virginia.
NASA has asked the Janus team to look at the feasibility of launching on the same rocket as NEO Surveyor in 2027, according to Dan Scheeres, the Janus principal investigator at the University of Colorado. With such a launch in 2027, Janus could capture the first up-close images of Apophis before RAMSES and OSIRIS-APEX get there.
“This is something that we’re currently presenting in some discussions with NASA, just to make sure that they understand what the possibilities are there,” Scheeres said in a meeting last week of the Small Bodies Advisory Group, which represents the asteroid science community.
“These spacecraft are capable of performing future scientific flyby missions to near-Earth asteroids,” Scheeres said. “Each spacecraft has a high-quality Malin visible imager and a thermal infrared imager. Each spacecraft has the ability to track and image an asteroid system through a close, fast flyby.”
“The scientific return from an Apophis flyby by Janus could be one of the best opportunities out there,” said Daniella DellaGiustina, lead scientist on the OSIRIS-APEX mission from the University of Arizona.
Binzel, who has led the charge for Apophis missions, said there is also some symbolic value to having a spacecraft escort the asteroid by Earth. Apophis will be visible in the skies over Europe and Africa when it is closest to our planet.
“When 2 billion people are watching this, they are going to ask, ‘What are our space agencies doing?’ And if the answer is, ‘Oh, we’ll be there. We’re getting there,’ which is OSIRIS-APEX, I don’t think that’s a very satisfying answer,” Binzel said.
“As the international space community, we want to demonstrate on April 13, 2029, that we are there and we are watching, and we are watching because we want to gain the most knowledge and the most understanding about these objects that is possible, because someday it could matter,” Binzel said. “Someday, our detailed knowledge of hazardous asteroids would be among the most important knowledge bases for the future of humanity.”
Armada to Apophis—scientists recycle old ideas for rare asteroid encounter Read More »