DNA-based bacterial parasite uses completely new DNA-editing method
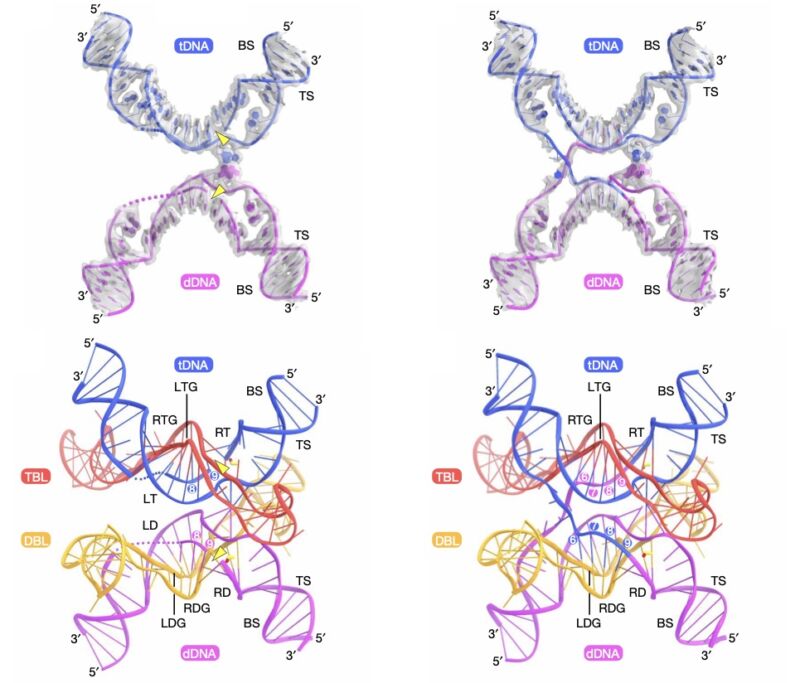
Enlarge / Top row: individual steps in the reaction process. Bottom row: cartoon diagram of the top, showing the position of each DNA and RNA strand.
Hiraizumi, et. al.
While CRISPR is probably the most prominent gene-editing technology, there are a variety of others, some developed before, others since. And people have been developing CRISPR variants to perform more specialized functions, like altering specific bases. In all of these cases, researchers are trying to balance a number of competing factors: convenience; flexibility; specificity and precision for the editing; low error rates; and so on.
So, having additional options for editing can be a good thing, enabling new ways of balancing those different needs. On Wednesday, a pair of papers in Nature describe a DNA-based parasite that moves itself around bacterial genomes through a mechanism that hasn’t been previously described. It’s nowhere near ready for use in humans, but it may have some distinctive features that make it worth further development.
Going mobile
Mobile genetic elements, commonly called transposons, are quite common in many species—they make up nearly half the sequences in the human genome, for example. They are indeed mobile, showing up in new locations throughout the genome, sometimes by cutting themselves out and hopping to new locations, other times by sending a copy out to a new place in the genome. For any of this to work, they need to have an enzyme that cuts DNA and specifically recognizes the right transposon sequence to insert into the cut.
The specificity of that interaction, needed to ensure the system only inserts new copies of itself, and the cutting of DNA, are features we’d like for gene editing, which places a value on better understanding these systems.
Bacterial genomes tend to have very few transposons—the extra DNA isn’t really in keeping with the bacterial reproduction approach of “copy all the DNA as quickly as possible when there’s food around.” Yet bacterial transposons do exist, and a team of scientists based in the US and Japan identified one with a rather unusual feature. As an intermediate step in moving to a new location, the two ends of the transposon (called IS110) are linked together to form a circular piece of DNA.
In its circular form, the DNA sequences at the junction act as a signal that tells the cell to make an RNA copy of nearby DNA (termed a “promoter”). When linear, each of the two bits of DNA on either side of the junction lacks the ability to act as a signal; it only works when the transposon is circular. And the researchers confirmed that there is in fact an RNA produced by the circular form, although the RNA does not encode for any proteins.
So, the research team looked at over 100 different relatives of IS110 and found that they could all produce similar non-protein-coding RNAs, all of which shared some key features. These included stretches where nearby sections of the RNA could base-pair with each other, leaving an unpaired loop of RNA in between. Two of these loops contained sequences that either base-paired with the transposon itself or at the sites in the E. coli genome where it inserted.
That suggests that the RNA produced by the circular form of the transposon helped to act as a guide, ensuring that the transposon’s DNA was specifically used and only inserted into precise locations in the genome.
Editing without precision
To confirm this was right, the researchers developed a system where the transposon would produce a fluorescent protein when it was properly inserted into the genome. They used this to show that mutations in the loop that recognized the transposon would stop it from being inserted into the genome—and that it was possible to direct it to new locations in the genome by changing the recognition sequences in the second loop.
To show this was potentially useful for gene editing, the researchers blocked the production of the transposon’s own RNA and fed it a replacement RNA that worked. So, you could potentially use this system to insert arbitrary DNA sequences into arbitrary locations in a genome. It could also be used with targeting RNAs that caused specific DNA sequences to be deleted. All of this is potentially very useful for gene editing.
Emphasis on “potentially.” The problem is that the targeting sequences in the loops are quite short, with the insertion site targeted by a recognition sequence that’s only four to seven bases long. At the short end of this range, you’d expect that a random string of bases would have an insertion site about once every 250 bases.
That relatively low specificity showed. At the high end, various experiments could see an insertion accuracy ranging from a close-to-being-useful 94 percent down to a positively threatening 50 percent. For deletion experiments, the low end of the range was a catastrophic 32 percent accuracy. So, while this has some features of an interesting gene-editing system, there’s a lot of work to do before it could fulfill that potential. It’s possible that these recognition loops could be made longer to add the sort of specificity that would be needed for editing vertebrate genomes, but we simply don’t know at this point.
DNA-based bacterial parasite uses completely new DNA-editing method Read More »