Kamikaze bacteria explode into bursts of lethal toxins
The needs of the many… —
If you make a big enough toxin, it’s difficult to get it out of the cells.
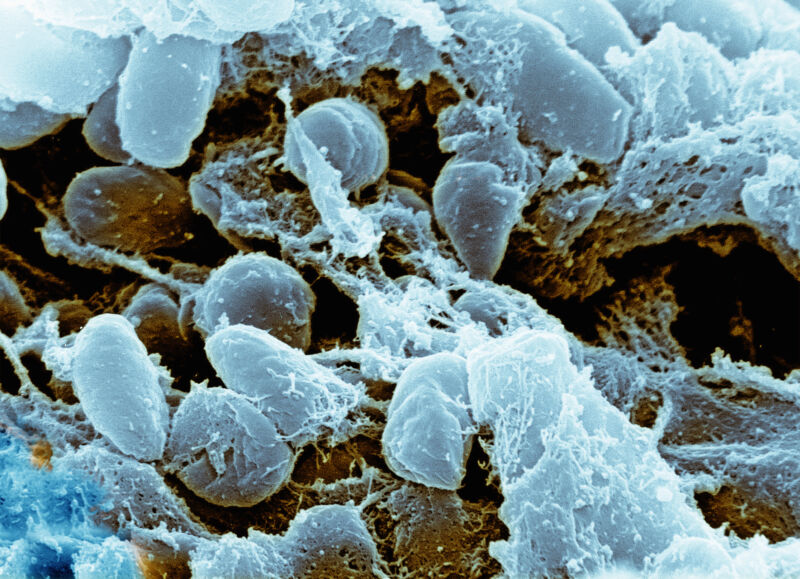
Enlarge / The plague bacteria, Yersina pestis, is a close relative of the toxin-producing species studied here.
Life-forms with no brain are capable of some astounding things. It might sound like sci-fi nightmare fuel, but some bacteria can wage kamikaze chemical warfare.
Pathogenic bacteria make us sick by secreting toxins. While the release of smaller toxin molecules is well understood, methods of releasing larger toxin molecules have mostly eluded us until now. Researcher Stefan Raunser, director of the Max Planck Institute of Molecular Physiology, and his team finally found out how the insect pathogen Yersinia entomophaga (which attacks beetles) releases its large-molecule toxin.
They found that designated “soldier cells” sacrifice themselves and explode to deploy the poison inside their victim. “YenTc appears to be the first example of an anti-eukaryotic toxin using this newly established type of secretion system,” the researchers said in a study recently published in Nature.
Silent and deadly
Y. entomophaga is part of the Yersinia genus, relatives of the plague bacteria, which produce what are known as Tc toxins. Their molecules are huge as far as bacterial toxins go, but, like most smaller toxin molecules, they still need to make it through the bacteria’s three cell membranes before they escape to damage the host. Raunser had already found in a previous study that Tc toxin molecules do show up outside the bacteria. What he wanted to see next was how and when they exit the bacteria that makes them.
To find out what kind of environment is ideal for Y. entomophaga to release YenTC, the bacteria were placed in acidic (PH under 7) and alkaline (PH over 7) mediums. While they did not release much in the acidic medium, the bacteria thrived in the high PH of the alkaline medium, and increasing the PH led it to release even more of the toxin. The higher PH environment in a beetle is around the mid-end of its gut, so it is now thought that most of the toxin is liberated when the bacteria reach that area.
How YenTc is released was more difficult to determine. When the research team used mass spectrometry to take a closer look at the toxin, they found that it was missing something: There was no signal sequence that indicated to the bacteria that the protein needed to be transported outside the bacterium. Signal sequences, also known as signal peptides, are kind of like built-in tags for secretion. They are in charge of connecting the proteins (toxins are proteins) to a complex at the innermost cell membrane that pushes them through. But YenTC apparently doesn’t need a signal sequence to export its toxins into the host.
About to explode
So how does this insect killer release YenTc, its most formidable toxin? The first test was a process of elimination. While YenTc has no signal sequence, the bacteria have different secretion systems for other toxins that it releases. Raunser thought that knocking out these secretion systems using gene editing could possibly reveal which one was responsible for secreting YenTc. Every secretion system in Y. entomophaga was knocked out until no more were left, yet the bacteria were still able to secrete YenTc.
The researchers then used fluorescence microscopy to observe the bacteria releasing its toxin. They inserted a gene that encodes a fluorescent protein into the toxin gene so the bacteria would glow when making the toxin. While not all Y. entomophaga cells produced YenTc, those that did (and so glowed) tended to be larger and more sluggish. To induce secretion, PH was raised to alkaline levels. Non-producing cells went about their business, but YenTc-expressing cells only took minutes to collapse and release the toxin.
This is what’s called a lytic secretion system, which involves the rupture of cell walls or membranes to release toxins.
“This prime example of self-destructive cooperation in bacteria demonstrates that YenTc release is the result of a controlled lysis strictly dedicated to toxin release rather than a typical secretion process, explaining our initially perplexing observation of atypical extracellular proteins,” the researchers said in the same study.
Yersinia also includes pathogenic bacteria that cause tuberculosis and bubonic plague, diseases that have devastated humans. Now that the secretion mechanism of one Yersinia species has been found out, Raunser wants to study more of them, along with other types of pathogens, to see if any others have kamikaze soldier cells that use the same lytic mechanism of releasing toxins.
The discovery of Y. entomophaga’s exploding cells could eventually mean human treatments that target kamikaze cells. In the meantime, we can at least be relieved we aren’t beetles.
Nature Microbiology, 2024. DOI: 10.1038/s41564-023-01571-z
Kamikaze bacteria explode into bursts of lethal toxins Read More »