Researchers find a new organelle evolving
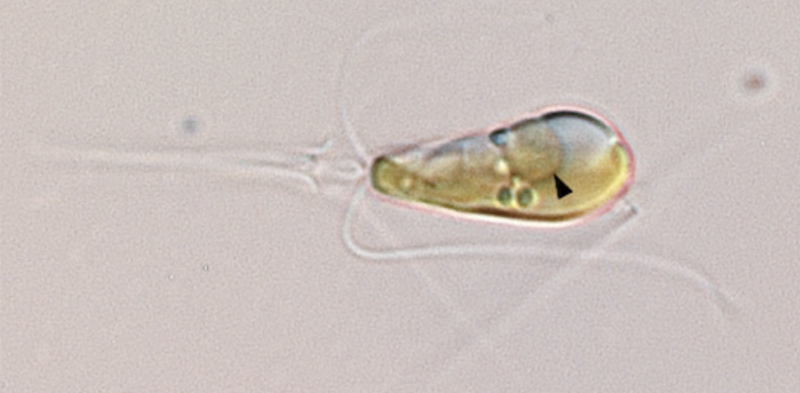
Enlarge / A photo of Braarudosphaera bigelowii with the nitroplast indicated by an arrowhead.
The complex cells that underlie animals and plants have a large collection of what are called organelles—compartments surrounded by membranes that perform specialized functions. Two of these were formed through a process called endosymbiosis, in which a once free-living organism is incorporated into a cell. These are the mitochondrion, where a former bacteria now handles the task of converting chemical energy into useful forms, and the chloroplast, where photosynthesis happens.
The fact that there are only a few cases of organelles that evolved through endosymbiosis suggests that it’s an extremely rare event. Yet researchers may have found a new case, in which an organelle devoted to fixing nitrogen from the atmosphere is in the process of evolving. The resulting organelle, termed a nitroplast, is still in the process of specialization.
Getting nitrogen
Nitrogen is one of the elements central to life. Every DNA base, every amino acid in a protein contains at least one, and often several, nitrogen atoms. But nitrogen is remarkably difficult for life to get ahold of. N2 molecules might be extremely abundant in our atmosphere, but they’re extremely difficult to break apart. The enzymes that can, called nitrogenases, are only found in bacteria, and they don’t work in the presence of oxygen. Other organisms have to get nitrogen from their environment, which is one of the reasons we use so much energy to supply nitrogen fertilizers to many crops.
Some plants (notably legumes), however, can obtain nitrogen via a symbiotic relationship with bacteria. These plants form specialized nodules that provide a habitat for the nitrogen-producing bacteria. This relationship is a form of endosymbiosis, where microbes take up residence inside an organism’s body or cells, with each organism typically providing chemicals that the other needs.
In more extreme cases, endosymbiosis can become obligatory. with neither organism able to survive without the other. In many insects, endosymbionts are passed on to offspring during the production of eggs, and the microbes themselves often lack key genes that would allow them to live independently.
But even states like this fall short of the situation found in mitochondria and chloroplasts. These organelles are thoroughly integrated into the cell, being duplicated and distributed when cells divide. They also have minimal genomes, with most of their proteins made by the cell and imported into the organelles. This level of integration is the product of over a billion years of evolution since the endosymbiotic relationship first started.
It’s also apparently a difficult process, based on its apparent rarity. Beyond mitochondria and chloroplasts, there’s only one confirmed example of a more recent endosymbiosis between eukaryotes and a bacterial species. (There are a number of cases where eukaryotic algae have been incorporated by other eukaryotes. Because these cells have compatible genetics, this occurs with a higher frequency.)
That’s why finding another example is such an exciting prospect.
Researchers find a new organelle evolving Read More »