TDK claims insane energy density in solid-state battery breakthrough
All charged up —
Apple supplier says new tech has 100 times the capacity of its current batteries.
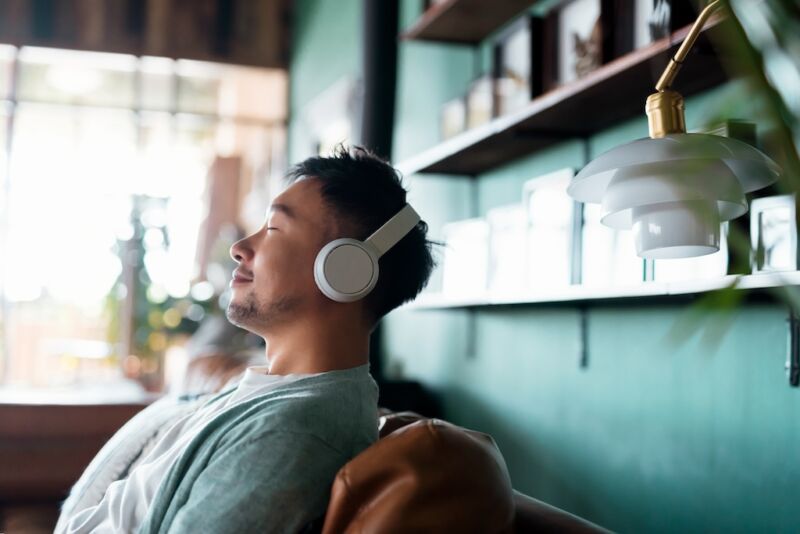
Enlarge / TDK says its new ceramic materials for batteries will improve the performance of small consumer electronics devices such as smartwatches and wireless headphones
Japan’s TDK is claiming a breakthrough in materials used in its small solid-state batteries, with the Apple supplier predicting significant performance increases for devices from wireless headphones to smartwatches.
The new material provides an energy density—the amount that can be squeezed into a given space—of 1,000 watt-hours per liter, which is about 100 times greater than TDK’s current battery in mass production. Since TDK introduced it in 2020, competitors have moved forward, developing small solid-state batteries that offer 50 Wh/l, while rechargeable coin batteries using traditional liquid electrolytes offer about 400 Wh/l, according to the group.
“We believe that our newly developed material for solid-state batteries can make a significant contribution to the energy transformation of society. We will continue the development towards early commercialisation,” said TDK’s chief executive Noboru Saito.
The batteries set to be produced will be made of an all-ceramic material, with oxide-based solid electrolyte and lithium alloy anodes. The high capability of the battery to store electrical charge, TDK said, would allow for smaller device sizes and longer operating times, while the oxide offered a high degree of stability and thus safety. The battery technology is designed to be used in smaller-sized cells, replacing existing coin-shaped batteries found in watches and other small electronics.
The breakthrough is the latest step forward for a technology industry experts think can revolutionize energy storage, but which faces significant obstacles on the path to mass production, particularly at larger battery sizes.
Solid-state batteries are safer, lighter and potentially cheaper and offer longer performance and faster charging than current batteries relying on liquid electrolytes. Breakthroughs in consumer electronics have filtered through to electric vehicles, although the dominant battery chemistries for the two categories now differ substantially.
The ceramic material used by TDK means that larger-sized batteries would be more fragile, meaning the technical challenge of making batteries for cars or even smartphones will not be surmounted in the foreseeable future, according to the company.
Kevin Shang, senior research analyst at Wood Mackenzie, a data and analytics firm, said that “unfavorable mechanical properties,” as well as the difficulty and cost of mass production, are challenges for moving the application of solid-state oxide-based batteries into smartphones.
Industry experts believe the most significant use case for solid-state batteries could be in electric cars by enabling greater driving range. Japanese companies are in the vanguard of a push to commercialize the technology: Toyota is aiming for as early as 2027, Nissan the year after and Honda by the end of the decade.
Car manufacturers are focused on developing sulfide-based electrolytes for long-range electric vehicles, an alternative kind of material to the oxide-based material that TDK has developed.
However, there is still skepticism about how quickly the much-hyped technology can be realized, particularly the larger batteries needed for electric vehicles.
Robin Zeng, founder and chief executive of CATL, the world’s biggest electric vehicle battery manufacturer, told the Financial Times in March that solid-state batteries did not work well enough, lacked durability and still had safety problems. Zeng’s CATL originated as a spin-off from Amperex Technology, or ATL, which is a subsidiary of TDK and is the world’s leading producer of lithium-ion batteries.
TDK, which was founded in 1935 and became a household name as a top cassette tape brand in the 1960s and 1970s, has lengthy experience in battery materials and technology.
It has 50 to 60 percent global market share in the small-capacity batteries that power smartphones and is targeting leadership in the medium-capacity market, which includes energy storage devices and larger electronics such as drones.
The group plans to start shipping samples of its new battery prototype to clients from next year and hopes to be able to move into mass production after that.
© 2024 The Financial Times Ltd. All rights reserved. Not to be redistributed, copied, or modified in any way.
TDK claims insane energy density in solid-state battery breakthrough Read More »