To kill the competition, bacteria throw pieces of dead viruses at them
Murderous —
A network of mutual murder ensures that diverse populations of bacteria survive.
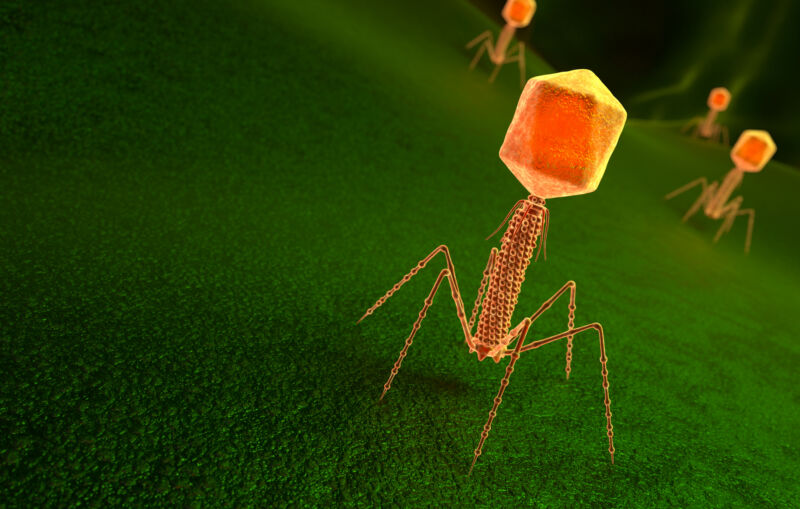
Enlarge / This is an intact phage. A tailocin looks like one of these with its head cut off.
Long before humans became interested in killing bacteria, viruses were on the job. Viruses that attack bacteria, termed “phages” (short for bacteriophage), were first identified by their ability to create bare patches on the surface of culture plates that were otherwise covered by a lawn of bacteria. After playing critical roles in the early development of molecular biology, a number of phages have been developed as potential therapies to be used when antibiotic resistance limits the effectiveness of traditional medicines.
But we’re relative latecomers in terms of turning phages into tools. Researchers have described a number of cases where bacteria have maintained pieces of disabled viruses in their genomes and converted them into weapons that can be used to kill other bacteria that might otherwise compete for resources. I only just became aware of that weaponization, thanks to a new study showing that this process has helped maintain diverse bacterial populations for centuries.
Evolving a killer
The new work started when researchers were studying the population of bacteria associated with a plant growing wild in Germany. The population included diverse members of the genus Pseudomonas, which can include plant pathogens. Normally, when bacteria infect a new victim, a single strain expands dramatically as it successfully exploits its host. In this case, though, the Pseudomonas population contained a variety of different strains that appeared to maintain a stable competition.
To learn more, the researchers obtained over 1,500 individual genomes from the bacterial population. Over 99 percent of those genomes contained pieces of virus, with the average bacterial strain having two separate chunks of virus lurking in their genomes. All of these had missing parts compared to a functional virus, suggesting they were the product of a virus that had inserted in the past but had since picked up damage that disabled them.
On its own, that’s not shocking. Lots of genomes (including our own) have plenty of disabled viruses in them. But bacteria tend to eliminate extraneous DNA from their genomes fairly quickly. In this case, one particular viral sequence appeared to date back to the common ancestor of many of the strains since all of them had the virus inserted at the same location of the genome, and all instances of this particular virus had been disabled by losing the same set of genes. The researchers termed this sequence VC2.
Many phages have a stereotypical structure: a large “head” that contains their genetic material, perched on top of a stalk that ends in a set of “legs” that help latch on to their bacterial victims. Once the legs make contact, a stalk contracts, an action that helps transfer the virus’ genome into the bacterial cell. In VC2’s case, all copies of it lacked the genes for producing the head section, as well as all the genes needed for processing its genome during infection.
This made the researchers suspect VC2 was something called a “tailocin.” These are former phages that have been domesticated by bacteria so they can be used to harm the bacteria’s potential competition. Bacteria with a tailocin can produce partial phages that consist only of the legs and stalk. These tailocins can still find and latch on to other bacteria, but when the stalk contracts, there’s no genome to inject. Instead, this just opens a hole in the membrane of their victim, partially eliminating the boundary of the cell and allowing some of its contents to leak out, leading to its death.
An evolutionary free-for-all
To confirm that the VC2 sequence encodes a tailocin, the researchers grew some bacteria that contained the sequence, purified proteins from it, and used electron microscopy to confirm that they contained headless phages. Exposing other bacteria to the tailocin, they found that while the strain that produced it was immune, many other strains growing in the same environment were killed by it. When the team deleted the genes that encode key parts of the tailocin, the killing went away.
The researchers hypothesize that the system is used to kill off potential competition but that many strains have evolved resistance to the tailocin.
When the researchers did a genetic screen to identify resistant mutants, they found that resistance was provided by mutations that interfered with the production of complex sugar molecules that are found on proteins that end up on the exterior of cells. At the same time, most of the genetic differences among the VC2 genes occur in the proteins that encode the legs, which latch on to these sugars.
So it appears that every bacterial strain is both an aggressor and a victim, and there’s an evolutionary arms race that leads to a complex collection of pairwise interactions among the strains—think of a rock/paper/scissors game with dozens of options. And the arms race has a history. Using old samples, the researchers show that many of the variations in these genes have been around for at least 200 years.
Evolutionary competitions are often viewed as a simple one-against-one fight, probably because it’s an easy way to think about them. But the reality is that most are more akin to a chaotic bar room brawl—one where it’s rare for any faction to obtain a permanent advantage.
Science, 2024. DOI: 10.1126/science.ado0713 (About DOIs).
To kill the competition, bacteria throw pieces of dead viruses at them Read More »