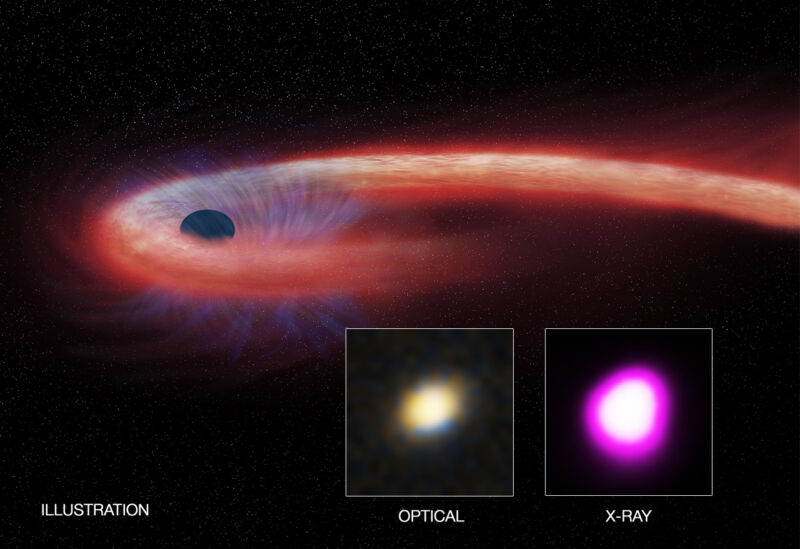
Enlarge / A model of a tidal disruption, along with some observations of one.
Supermassive black holes appear to be present at the core of nearly every galaxy. Every now and again, a star wanders too close to one of these monsters and experiences what’s called a tidal disruption event. The black hole’s gravity rips the star to shreds, resulting in a huge burst of radiation. We’ve observed this happening several times now.
But we don’t entirely know why it happens—”it” specifically referring to the burst of radiation. After all, stars produce radiation through fusion, and the tidal disruption results in the spaghettification of the star, effectively pulling the plug on the fusion reactions. Black holes brighten when they’re feeding on material, but that process doesn’t look like the sudden burst of radiation from a tidal disruption event.
It turns out that we don’t entirely know how the radiation is produced. There are several competing ideas, but we’ve not been able to figure out which one of them fits the data best. However, scientists have taken advantage of an updated software package to model a tidal disruption event and show that their improved model fits our observations pretty well.
Spaghettification simulation
As mentioned above, we’re not entirely sure about the radiation source in tidal disruption events. Yes, they’re big and catastrophic, and so a bit of radiation isn’t much of a surprise. But explaining the details of that radiation—what wavelengths predominate, how quickly its intensity rises and falls, etc.—can tell us something about the physics that dominates these events.
Ideally, software should act as a bridge between the physics of a tidal disruption and our observations of the radiation they produce. If we simulate a realistic disruption and have the physics right, then the software should produce a burst of radiation that is a decent match for our observations of these events. Unfortunately, so far, the software has let us down; to keep things computationally manageable, we’ve had to take a lot of shortcuts that have raised questions about the realism of our simulations.
The new work, done by Elad Steinberg and Nicholas Stone of The Hebrew University, relies on a software package called RICH that can track the motion of fluids (technically called hydrodynamics). And, while a star’s remains aren’t fluid in the sense of the liquids we’re familiar with here on Earth, their behavior is primarily dictated by fluid mechanics. RICH was recently updated to better model radiation emission and absorption by the materials in the fluid, which made it a better fit for modeling tidal disruptions.
The researchers still had to take a few shortcuts to ensure that the computations could be completed in a realistic amount of time. The version of gravity used in the simulation isn’t fully relativistic, and it’s only approximated in the area closest to the black hole. But that sped up computations enough that the researchers could track the remains of the star from spaghettification to the peak of the event’s radiation output, a period of nearly 70 days.