We still don’t understand how one human apparently got bird flu from a cow
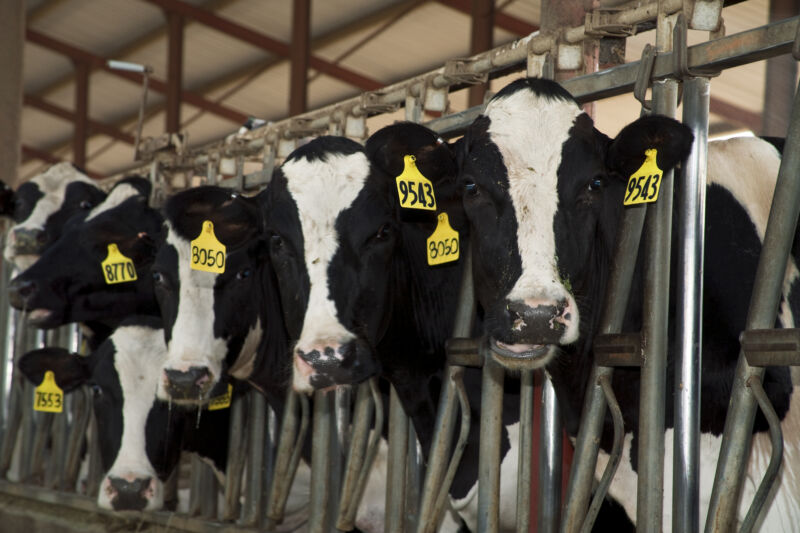
Enlarge / Holstein dairy cows in a freestall barn.
The US Department of Agriculture this week posted an unpublished version of its genetic analysis into the spillover and spread of bird flu into US dairy cattle, offering the most complete look yet at the data state and federal investigators have amassed in the unexpected and worrisome outbreak—and what it might mean.
The preprint analysis provides several significant insights into the outbreak—from when it may have actually started, just how much transmission we’re missing, stunning unknowns about the only human infection linked to the outbreak, and how much the virus continues to evolve in cows. The information is critical as flu experts fear the outbreak is heightening the ever-present risk that this wily flu virus will evolve to spread among humans and spark a pandemic.
But, the information hasn’t been easy to come by. Since March 25—when the USDA confirmed for the first time that a herd of US dairy cows had contracted the highly pathogenic avian influenza H5N1 virus—the agency has garnered international criticism for not sharing data quickly or completely. On April 21, the agency dumped over 200 genetic sequences into public databases amid pressure from outside experts. However, many of those sequences lack descriptive metadata, which normally contains basic and key bits of information, like when and where the viral sample was taken. Outside experts don’t have that crucial information, making independent analyses frustratingly limited. Thus, the new USDA analysis—which presumably includes that data—offers the best yet glimpse of the complete information on the outbreak.
Undetected spread
One of the big takeaways is that USDA researchers think the spillover of bird flu from wild birds to cattle began late last year, likely in December. Thus, the virus likely circulated undetected in dairy cows for around four months before the USDA’s March 25 confirmation of an infection in a Texas herd.
This timeline conclusion largely aligns with what outside experts previously gleaned from the limited publicly available data. So, it may not surprise those following the outbreak, but it is worrisome. Months of undetected spread raise significant concerns about the country’s ability to identify and swiftly respond to emerging infectious disease outbreaks—and whether public health responses have moved past the missteps seen in the early stages of the COVID-19 pandemic.
But another big finding from the preprint is how many gaps still exist in our current understanding of the outbreak. To date, the USDA has identified 36 herds in nine states that have been infected with H5N1. The good news from the genetic analysis is that the USDA can draw lines connecting most of them. USDA researchers reported that “direct movement of cattle based upon production practices” seems to explain how H5N1 hopped from the Texas panhandle region—where the initial spillover is thought to have occurred—to nine other states, some as far-flung as North Carolina, Michigan, and Idaho.
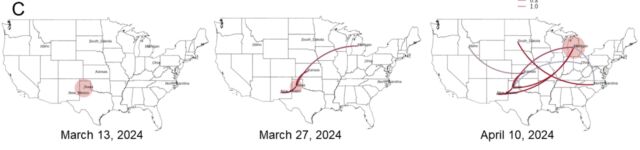
Enlarge / Bayes factors for inferred movement between different discrete traits of H5N1 clade 2.3.4.4b viruses demonstrating the frequency of movement.
Enlarge / Putative transmission pathways of HPAI H5N1 clade 2.3.4.4b genotype B3.13 supported by epidemiological links, animal movements, and genomic analysis.
Putative transmission pathways of HPAI H5N1 clade 2.3.4.4b genotype B3.13 supported by epidemiological links, animal movements, and genomic analysis. [/ars_img]The bad news is that those lines connecting the herds aren’t solid. There are gaps in which the genetic data suggests unidentified transmission occurred, maybe in unsampled cows, maybe in other animals entirely. The genetic data is clear that once this strain of bird flu—H5N1 clade 2.3.4.4 genotype B3.13 —hopped into cattle, it could readily spread to other mammals. The genetic data links viruses from cattle moving many times into other animals: There were five cattle-to-poultry jumps, one cattle-to-raccoon transmission, two events where the virus moved from cattle to domestic cats, and three times when the virus from cattle spilled back into wild birds.
“We cannot exclude the possibility that this genotype is circulating in unsampled locations and hosts as the existing analysis suggests that data are missing and undersurveillance may obscure transmission inferred using phylogenetic methods,” the USDA researchers wrote in their preprint.
We still don’t understand how one human apparently got bird flu from a cow Read More »